Category: audio
B&O Tech: Where should I decode?
#10 in a series of articles about the technology behind Bang & Olufsen loudspeakers
One question that I am occasionally asked is why the BeoVision 11 is not able to decode audio signals encoded in Dolby TrueHD and DTS HD-Master Audio. I am not able to answer this question. However I can discuss what the implications are with respect to audio and, more specifically, audio quality in a playback system.
Before we start this discussion, let’s get a couple of terms defined:
- LPCM: Linear Pulse Code Modulation. This is what most people call “uncompressed digital audio”. It’s the way digital music is stored on a CD, for example. It’s also the way digital audio is encoded to be able to send it around a computer or digital signal processor and do things like filter it, mix it, or just change the volume. So, at some point, in any system that has any digital audio signals anywhere in it (even if it’s just to change the volume, for example), it will be sending the signals around as LPCM-encoded audio.
- CODEC: COmpression-DECompression. This is a way of encoding the LPCM audio signal so that it takes up less space (or less bandwidth). This is the audio equivalent of converting something to a “ZIP” file – sort of. Some CODEC’s are “lossless” – meaning that, if you take a signal, compress it and decompress it, you get back everything you put in – a bit-for-bit match of the original. (If you didn’t, it wouldn’t be “lossless”). Other CODEC’s are “lossy” – meaning that, when you compress the signal, some stuff is thrown away (this is why audio professionals call lossy CODEC’s like MP3 “data reduction” instead of “audio compression”). Hopefully, the stuff that’s thrown away is stuff you can’t hear – but that debate is best left out of this discussion.
- Bitstream: Some Blu-ray players allow you to choose whether you send the audio data that’s on the disc directly out its digital output, unchanged OR to decode the audio data to LPCM before sending it out. Typically, this shows up in the menus on the player as a choice between sending “bitstream” or “LPCM”. So, for the purposes of this article, I’ll use these two terms as meaning those two things. However, if we’re going to be accurate, this is an incorrect definition, since an LPCM signal is a stream of bits, and therefore is also a bitstream. But now I’m being purposely punctilious…
Let’s start this discussion by building a fairly standard home theatre system. We have:
- A Blu-ray player connected to…
- A BeoVision or BeoPlay television or a BeoSystem X OR some other brand’s AVR (Audio Video Receiver) or Surround Processor via an HDMI cable
which is connected to… - A surround sound setup with 5 or 7 main loudspeakers (some of those might be inside the television) and maybe a subwoofer, all connected to the television using power amp, or line level (or PowerLink, for B&O customers) connections
Let’s also assume the following:
- The Blu-ray player is connected to the television’s input with an HDMI cable.
- Both the Blu-ray player and the television (or AVR or Surround Processor) have been certified by Dolby and DTS to decode all the encoding formats in which we’re interested for this discussion
(note that this is not true of all devices in the real world – but we’ll use the assumption for now). - The player does what you tell it to do. In other words, if you set its output to bitstream, it sends the stream of bits that are on the disc it’s playing – and nothing else.
The question is:
What format should I use to send the audio signals from the Blu-ray player to the television? Should I set my Blu-ray player to send a Bitstream to the television and decode the audio signals there? Or maybe it’s better to tell the Blu-ray player to decode the signals and send LPCM to the television.
The answer, unfortunately, is potentially complicated… but let’s look at what the implications are for the two options.
Audio Quality
If you do a search on the web, you’ll find all sorts of answers to this question. Some of the answers are correct, some are partly correct, and some are just plain wrong – actually, some aren’t even wrong.
If you go to a reasonably reputable source such as www.hdmi.org, you’ll read that “There is no inherent difference in quality between Dolby TrueHD/DTS-HD being sent over HDMI as decoded PCM vs. encoded bit stream“. This is, in fact, true (although it’s not the full story). It’s true if your audio is encoded in Dolby TrueHD, Dolby Digital Plus, Dolby Digital, DTS-HD Master Audio, DTS-HD High Resolution Audio, or DTS Digital Surround.
The basic reason for this is that Bang & Olufsen, like every other company that manufacturers audio/video components that support these formats must adhere to a very strict set of regulations that are set by Dolby and DTS in order to receive certification for our products. Also, before we can deliver a new product to our dealers, it must be thoroughly tested by both Dolby and DTS to ensure that we meet their exacting standards for their formats. In other words, a Dolby TrueHD decoder is a Dolby TrueHD decoder – regardless of what the box it’s in looks like.
This means that every device that is certified to decode Dolby TrueHD or DTS-HD Master Audio and convert that format to an uncompressed PCM audio signal (footnote: the “standard” format that is used by the internal Digital Signal Processing (DSP) of the device) does that decoding in the same way. This is true whether the device is a Blu-ray player, a DVD player, an AVR, or surround sound processor. Note that this does NOT, however, mean that a loudspeaker connected to any of these devices will give you the same sound quality – there are many, many other links in the audio chain that have an effect on sound. It merely means that the conversion from a compressed (either lossy or lossless) signal to an uncompressed signal will be identical, regardless of where it’s done.
So, whether:
- you decode in the player and send PCM to the television, OR
- you send the bitstream to the television and decode the signal there,
there is no difference in audio quality
Now, you might tell me “But I went to a user forum and saw a posting from a person who did a test with his Acme Blu-ray player and his Flybynight AVR and he reports that he can EASILY hear the difference in quality in the audio when he changes from LPCM to Bitstream on his player.” This may, in fact, be true. However, the reason that there is an audible difference is not due to one of the devices having a better decoder or some very minor issue like jitter on the HDMI signal. There are at least two very basic and very simple explanations as to why this might happen.
- The first is a simple level difference. If you do an ABX test of a system where A or B is 1 dB louder than the other, but are identical in every other aspect, your listening test subjects will have no difficulty identifying what X is. (An ABX test is one where you can listen to 3 things – an “A”, a “B” and an “X”. “X” is guaranteed to be identical to either “A” or “B” (which are guaranteed to be different). Your task is to identify whether “X” matches “A” or “B”. So, if the Bitstream is just 1 dB (or less!) louder than than the LCPM version, then you will hear a difference – although you might not notice that the difference is a simple loudness. It’s likely that you will perceive the louder one as just being “better”.
- The second is that some AVR’s can be set to apply different post processing to LPCM signals than they do to signals decoded internally. So, it’s possible that there could be something as simple as a bass or treble difference between the two signals – but it could be something as complicated as a lot of spatialisation and reverberation with a big EQ curve – or anything in-between. So, in this case, the difference between Bitstream and LPCM coming in from the player is in the post-processing differences in the AVR.
However, as I said, this is not the full story. Let’s look at more pieces of the puzzle to see what the differences are.
Audio during fast-forward / fast-rewind
Different players behave differently when you are fast-forwarding or fast-rewinding through a disc. If you are sending an encoded bitstream from the player, many devices will not deliver any audio when you are moving through your disc at a higher speed. This behaviour is different from brand to brand and model to model. However, in many cases, these same players WILL deliver audio if the output is set to PCM.
Listening Levels
In a theory, there should be no difference in audio levels (i.e. how loud the signals are) caused by moving from a decoder inside your player to one inside your television. However, as I mentioned above, this world isn’t perfect – and one of the results of that imperfection is that there may, indeed, be a difference in level caused by switching from a bitstream to a PCM output from the Blu-ray player. If this does happen, then it’s likely because of some extra (or different) post-processing that is happening to the signal(s).
Mixing of Extra Audio Channels
One of the cool features of DVD and Blu-ray (that I, personally, never use) is that you can watch a movie whilst listening to the director (or someone else) talk about the movie – a feature usually called “Commentary” or something like that. This is fun, because there’s nothing that can make IronMan 3 more interesting than hearing about how someone accidentally spilled a cup of coffee on their computer keyboard while they were rendering a 3D CGI version of an Audi falling into the ocean.
In order for this feature to work, the system has to take the audio signals for the movie and mix in an additional audio channel. However, some players are not able to mix these two sounds together if they’re outputting a bitstream. They can only do it if they’re decoding the movie AND the commentary separately, and then mixing the two LPCM streams. Of course, to then re-encode the result, just to send out a bitstream would be silly.
Another example of these extra sounds that may not make it through a bitstream output are the “clicks” or cute noises that are assigned to menu items.
So, with some players, if you want to hear these extra sounds, you’ll have to output a decoded LPCM stream.
Channel Allocation and Routing
This is where things get a little debatable. Usually, when people say “5.1” they mean the following channel allocations (in no particular order)
- Left Front
- Centre Front
- Right Front
- Left Surround
- Right Surround
- LFE
And, when they say “7.1” they mean
- Left Front
- Centre Front
- Right Front
- Left Surround
- Right Surround
- Left Back
- Right Back
- LFE
We’ll call these the “standard audio channel allocations” for this article. However, as I talked about it a previous article, “7.1” actually has seven “legal” variants that include non-standard audio channel allocations like front-wide and height channels.
Let’s assume, temporarily, that you have a disc that has some audio channels on it that should be routed to, say, a ceiling loudspeaker. The question is: “IF your surround processor has a “ceiling speaker” output, and IF there is a “ceiling” audio channel on the disc, can the signal get to the correct loudspeaker?” The answer is complicated. . .
Firstly, the question is “how can the disc ‘know’ that the audio channel is a ‘ceiling’ channel?” Well, both Dolby TrueHD and DTS HD-Master Audio (for example) have the option to include “metadata” (this is a fancy word meaning “data about the data” – or, in other words “information about the audio signals”) that can tell the decoder something like “audio channel #6 should be sent to a ceiling loudspeaker”. Other CODEC’s (like Dolby Digital, for example) do not have the possibility to have non-standard audio
Secondly, the question is “if the player decodes the signal to LPCM, and its decoder knows that the channel should be routed to the ceiling, can it ‘tell’ the surround processor via the HDMI metadata where to route the signal?” The answer to this question is dependent on the version numbers of the HDMI transmitter in the player and the HDMI receiver in the surround processor. If you are using HDMI 1.3 or earlier, then you cannot have non-standard channel allocations with an LPCM signal. If you have HDMI 1.4 or higher (for both the transmitter and the receiver) you might be able to get the correct metadata across from device to device. (if you look here, you can see that, if your HDMI transmitter or receiver is version 1.2 or earlier, then you cannot send the Dolby or DTS lossless codec’s – so this will also not work for non-standard channel allocations.)
So, the only way to guarantee that your complete system can support non-standard audio channel allocations (assuming that your surround processor has the ability to output them) is to send a bitstream from the player and decode at the end of the chain.
However, the question to ask after you’ve answered all of that is “how many commercially-available recordings include non-standard audio channel allocations?” The answer to this, as far as I’ve been able to figure out is “none”. (If you know of any examples of this that prove me wrong, please leave a note in the “replies” – I’m looking for materials! But read the rest of this paragraph first…) Of course, there are SACD’s where the LFE channel should be directed to a height channel – but SACD’s don’t include metadata to tell the player about the routing. Dolby ProLogic IIz and dts Neo:X have height channel outputs, but that’s a different system than a CODEC with discrete output channels. There are some other formats like Imax, Auro3D, and Dolby Atmos that use height channels, but they’re not available in consumer media.
So, this, at least for now, is an solution without a problem.
Bass Management
Some Blu-ray players (and even some good ol’ DVD players) include a bass management system that will filter the bass out of the main channels and add it to the LFE output.
IF your player can do this, then:
- it can only do it to a decoded signal – so the bass management in the player will not work with a bitstream output
- you should be sure that it is doing it (if you want it to do so) or that it is not doing it (if you don’t)
Latency
Some forum discussion groups have highlighted an issue with some specific players that exhibit lip-synch problems when they decode the signal to LPCM internally. The few comments about this that I have read in these fora indicate that switching the player’s output to bitstream appears to fix this problem. However, this should be considered a “work-around” for a bug in the player’s software. There should be no difference in synchronisation of sound and picture whether you’re decoding in the player or the surround processor. If there is a lip synch problem, then the people that made the player haven’t done their jobs properly.
Conclusion
So, to wrap up: the big things to remember here are that,
- in any audio playback system, audio that is stored (and/or transmitted) in a CODEC has to be converted to LPCM somewhere
- there is no difference in quality of decoder – in other words a Dolby (or DTS) decoder in one device won’t be better than a Dolby (or DTS) decoder in another device. If it were, then Dolby (or DTS) would not have approved one of them
- there are some issues not related to audio quality that are affected by the location of the decoder in an audio chain, but these are typically very small (or even non-existent) issues for almost all consumers
Finally, I didn’t talk about jitter – which is term that a lot of people throw around as being one of those evils in every audio system where you can place all of your blame for everything that is bad about the system. This puts it in a league with things like “society“, “television“, “Canada“, and “The Boogie“. I’ll talk about that sometime in the future.
One last thing
Everything I’ve said above is only true for an HDMI connection. If you have an S/P-DIF or TOSLINK connection, then the discussion will be quite different, since you cannot have more than 2 channels of LPCM-encoded audio on those systems – so the only way to get multichannel audio through it is to use a lossy CODEC. So, if you have a multichannel source and you decode to LPCM before sending it out on S/P-DIF or TOSLINK, you will wind up with a 2.0 channel downmix.
Reports of its death have been greatly exaggerated
B&O Tech: Thermal Compression Compensation
#9 in a series of articles about the technology behind Bang & Olufsen loudspeakers
Recipe for
`Befuddled Speaker Enthusiast´
Makes: One individual with reduced faith in loudspeaker reviews
Total time: approximately 2 hours
Directions
- Take a woofer and put it in a cabinet
- Connect an amplifier to it
- Put it in a sauna
- Set the room temperature to 20° C and wait until everything in the room is the same temperature
- Measure the woofer’s on-axis response with a microphone
- Look at the pretty plot of its magnitude response
- Turn up the thermostat to 100° C and wait until the woofer warms up
- Measure the response again
- Look at the new pretty plot of its magnitude response
- Scratch your head while you ask yourself why the two measurements look so different.
The setup
When you read a magazine review of a loudspeaker, it will include a measurement of its “frequency response” (more accurately called its “magnitude response”) which shows (ignoring a bunch of things) how loud different frequencies are when they come out of the loudspeaker assuming that they all came in at the same level.
However, as we saw in a previous article, for a Bang & Olufsen loudspeaker, this magnitude response is dependent not only on the loudspeaker, but how loudly you’re playing the signal.
Unfortunately, it gets much worse than this… For example, if we take a woofer (say, the one from the recipe above, for example) we can explain its electromechanical characteristics by breaking it down into different components (both actual and analogical). For example, the suspension (which is comprised of the surround and the spider) can be thought of as a spring. The electrical analogy for this is a capacitor. If you take all of the moving parts in the loudspeaker driver, they all add up to a mass that has to be moved – the electrical analogy for that mass is an inductor (since an inductor has some electrical “inertia” just like the mass of a bunch of loudspeaker bits). Some of the components are not an electrical analogy – they are real electrical components. For example, the voice coil, since it’s a coil, acts as an inductor. And, since it is a thin bit of wire, it also has some resistance to the flow of electrical current through it, so it’s also a resistor.

If you look at the diagram above, you’ll see a very simplified “circuit” that shows the components of a moving coil dynamic loudspeaker. If these components don’t look familiar to you, don’t worry, it’s not important. Some components in the circuit are actual electrical things (like the resistance of the voice coil, shown in red) and others are analogies – electrical representations for a mechanical component in the system (such as a capacitor representing the “spring” of the surround and the spider).
If you know how each of these components behaves, and you know the correct values to put in for a given loudspeaker, and you know how to do the right math, then you can come pretty close to getting a decent prediction of the response of the loudspeaker that you’re modelling with the circuit. However, if you just put in one value for each component, then you’re assuming that they never change – in other words that you’re dealing with a “linear” system.
The problem is that this assumption is incorrect. For example, the Voice Coil Resistance – the amount that the wire in the voice coil resists the flow of current through it when the loudspeaker driver is not moving – changes with temperature. The hotter the wire gets, the higher the resistance goes. (This is a normal behaviour for most resistors.) If the voice coil resistance changes, then the whole system behaves differently, since it isn’t the only component in the circuit. So, as we change the temperature of the voice coil, the total response of the loudspeaker changes.
Sadly, the temperature of the voice coil isn’t only dependent on the room temperature as it seemed to be in our recipe for a Befuddled Speaker Enthusiast. As soon as you start playing sound with a loudspeaker, it starts heating up. The louder the signal (either because you turned up the volume or because your Metallica album just came on) the hotter it gets. So as you play music, it heats and cools. The speed with which it heats up and cools down is dependent on its “thermal time constant” – a big woofer with a giant magnet will take longer to heat up and cool down (and therefore have a longer thermal time constant) than a little tiny tweeter.
So, now you should have at least three questions that deserve answers:
- How much does the temperature vary when I play music?
- How does the response of the loudspeaker change with temperature?
- How much does the response of the loudspeaker change with temperature?
- What are you going to do about it?
1. Voice coil temperature
As I’ve talked about in a previous article, a loudspeaker driver is, give or take, about 1% efficient. That means that 99% of the power that you push into it (from the amplifier) is not converted into sound. Unfortunately, all of that power is lost as heat – almost all of it at the voice coil of the loudspeaker. So, the louder your music, the hotter your voice coil gets. Of course, if you have a way of cooling it (by using other parts of the loudspeaker as a radiator to your listening room) then it won’t get as hot, and it will cool down faster.
Let’s take a BeoLab 5 as an example (since that’s where we’re headed anyway…). Let’s take some relatively new-ish pop music (which has been mastered to be fairly loud due to a war that has been going on for years) and play it on a B&O player through Power Link (B&O’s version of a line level signal) at maximum volume on a BeoLab 5 whilst monitoring the temperature of the voice coils. What you’ll see if you do this is something like the plot below.
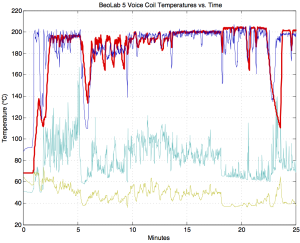
As you can see in the screenshot in Figure 2, the lower woofer (a 15″ driver connected to a 1000 W Ice Power amplifier) varied with a maximum of about 205° C. While it was playing this music at this level, it rarely dropped below 120°C.
This means that the difference in temperature of the woofer was 185°C at a maximum (205°C – 20°C) and rarely below 100°C.
In case you are wondering, this temperature cannot be measured directly, since it would destroy the voice coil if we tried to do so. Instead, what we do is to measure the temperature of the loudspeaker driver magnets, and use that real-time data input in addition to the signal that we’re sending to the drivers to calculate the temperatures of the voice coils based on thermal models of each of them. As you can see in Figure 3, below, the magnet temperatures are very different, and react much more slowly. These measurements were taken at exactly the same time as the ones shown in Figure 2. (Note that, although the mid woofer and woofer voice coils are roughly the same temperature, the mid woofer magnet is hotter than the woofer magnet by about 20°C or so. This just proves that their thermal models are different.)

2 & 3. Loudspeaker response changes
So, now the question is “what does this change in temperature do to the response of the driver?” We’ll only deal with one driver – the woofer.
As I mentioned above, the thing that changes most in the model shown in Figure 1 is the loudspeaker driver’s voice coil resistance. For those of you with a background in reading electrical circuits, you may notice that the one shown in Figure 1 has some reactive components in it which will result in a resonance at some frequency. For those of you without a background in reading electrical circuits, what this means is that a loudspeaker driver (like a woofer) has some frequency that it “wants” to ring at – if you thump it with your thumb, that’s the frequency that you will hear ringing – a bit like a bell with a low pitch.
As the voice coil resistance goes up, its resistance increases, and we generally lose sensitivity (i.e. level or loudness) from the woofer. In other words, the hotter it gets, the quieter it gets. However, this only happens at the frequencies where the resistor is not “overridden” by another component – say the mechanical resonance of the woofer or the inductance of the voice coil.
The total result is shown for various temperature differences in Figure 4. Notice that these plots show the change in magnitude response of the driver with CHANGES in temperature. So, the blue curve at the top is the change in magnitude response (which is 0 dB at all frequencies – in other words no change) when the loudspeaker is playing at the same temperature it was measured at (let’s say, 20°C or room temperature). As the temperature of the voice coil increases above that temperature, you can see that you lose output in two frequency bands on either side of a “bump” in the response – this is at the resonant frequency of the loudspeaker driver.
So, the louder you play, the more low end you lose, apart from a peak in the response (which also rings in time) at the resonant frequency of the driver.
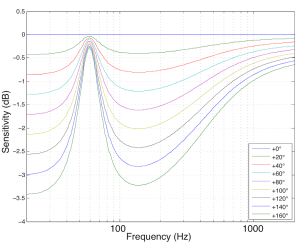
In case you’re wondering, the plot shown in Figure 4 is pretty close to the actual response of the 15″ woofer in the BeoLab 5 at different temperatures above room temperature.
The solution
Interestingly, all of the stuff I said above is true for every loudspeaker. So, if you’re the kind of person who believes that the only proper loudspeaker is one where you have nothing but a loudspeaker driver (in a cabinet of any kind, or not) and an amplifier – and no weird filtering or mucking-about going on, then you’ll have to live with the kind of unpredictable behaviour that you see above. This happens all the time to every dynamic loudspeaker. Since, in a passive loudspeaker, there’s nothing you can do about this (except for trying to keep the drivers cool somehow) you don’t often hear passive loudspeaker manufacturers talking about this little skeleton in their closet…
However, since a BeoLab 5 “knows” the temperature of the voice coil of the woofer, and since it has been programmed with the curves shown above in Figure 4, we can do something about it.
In essence all we need to do is to take Figure 4, flip it upside down and make a filter that “undoes” the effect of temperature on the loudspeaker’s response. In other words, if (because the woofer gets 160°C above “normal”) it drops 3 dB at 20 Hz, the BeoLab 5 knows this and adds 3 dB at 20 Hz. So, built into the BeoLab 5 is a set of filters that are used, depending on the temperature of the woofer’s voice coil. These filters are shown in Figure 5.
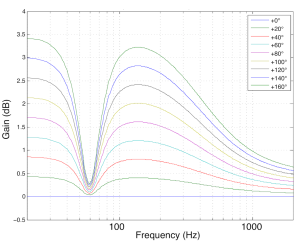
It’s important to note three things here.
- This can be done because we know the behaviour of the woofer at different temperatures (this was measured as part of the development process)
- This can be done because the loudspeaker “brain” (the DSP) knows the temperature of the voice coil in real time as you’re playing music
- This filter should only be applied to the woofer. The mid woofer and the other drivers have different behaviours and should not be affected by this correction curve. Therefore, this filtering can only be done because the BeoLab 5 is an active loudspeaker with independent filtering for each loudspeaker driver.
Some extra information
You should be left with at least one question. I said above that, as the music gets loud, the woofer heats up, so you lose output, so we add a filter that compensates by putting more signal into the driver.
“Waitaminute!” I hear you cry… “The problem is caused by the signal being too loud, so you make it louder!?” Well… yes.
However, there is one more trick up our sleeve. In a previous posting, I mentioned in passing that we also have Thermal Protection in almost all of the loudspeakers in the B&O portfolio. This means that the DSP brain knows the temperature of the drivers and, in a worst-case situation, turns the levels down to protect things from burning up. So, if we go back to the example of a BeoLab 5 playing at full volume, let’s see what’s happening to the signal levels.

These curves in Figure 6 show the gains applied to the four loudspeaker drivers in a BeoLab 5 at the same time as the measurements shown in Figures 2 and 3 were being made. In fact, if you look carefully at Figure 2 around the 23 minute mark, you’ll see that the temperature dropped – which is why the gain in Figure 6 increases (because it can!) in response.
Now, don’t panic. The BeoLab 5 isn’t screwing around with the gains of the drivers all the time. Remember that this test was done at FULL VOLUME – which, for a BeoLAb 5 is VERY LOUD. The gains shown in Figure 6 are a last-ditch effort of the loudspeaker to protect itself from a very mean customer (or the very mean children of a customer who is away for the weekend). This is the equivalent of the airbags deploying in your car. You know that if the airbags are outside the steering wheel (or if the gains in the BeoLab 5 dropped by 15 dB or so) something significant occurred…
Thanks to Gert Munch for his help in cleaning up the mistakes I made in the drafts up to and including the penultimate version of this article.
First Impressions
I’m sitting and listening to Mary Chapin Carpenter’s new album “Songs From the Movie” for the first time on Spotify on a pair of headphones.
I listen to a lot of recordings – usually to find problems in loudspeakers, so it’s not very often that an album makes the hair on the back of my neck stand up.
This one does.
I haven’t yet been able to find out who the recording engineer(s) was(were) for this album, but to whomever it was – Thanks!
B&O Tech: Subwoofer Tweaking for Beginners
#8 in a series of articles about the technology behind Bang & Olufsen loudspeakers
In a previous post, I talked about why a subwoofer might be a smart addition to a sound system – and why a subwoofer brings something different to a Bang & Olufsen loudspeaker configuration than it does for other companies’ loudspeakers.
Usually, in a loudspeaker system that includes a subwoofer, the signal that is sent to that subwoofer is either
- coming in directly from the medium (say, the Blu-ray disc) from the LFE (Low Frequency Effects) channel OR
- created by something called a “bass management system” which is basically a mixer (something that adds audio signals) and some frequency division OR
- all of the above
Let’s assume, for the purposes of this article, that we’re talking about #3. So, let’s start by talking about how a system like that would work. The simple version is that you take an audio input, send it to two different filters, one called a “high pass filter” which lets the high frequencies pass through it and it makes the lower frequencies quieter. The second filter is called a “low pass filter” – you can figure that one out. The output of the high pass filter is sent to your “main” loudspeaker, and the output of the low pass filter is sent to the subwoofer.
That’s what happens if you have a good ol’ fashioned monophonic system with only one audio channel, one main loudspeaker and one subwoofer. Most people nowadays, however, have more than one main loudspeaker and lots of channels coming out of their players. So, in cases like that, we have to take the low end (the bass) out of each of the main channels using low pass filters, add the results all together, add the LFE channel to that, and send the total to the subwoofer. A simple version (with some important details left out, since we’re only talking about basic concepts here…) is shown in the diagram below.
Basics of Signal Addition
Let’s take an audio signal and add it to another audio signal – and, just to keep things simple, we’ll make them both sine waves. This is done by looking at the amplitude of the two signals at a given moment in time, adding those two values, and you get a result. For example:
If you take a look at the example above, there are a couple of things that you can see. The first is that, if you add two sine waves, you get a sine wave. Also, if you add two sine waves of the same frequency, you get a sine wave of the same frequency. Next, if the two sine waves have the same amplitude and are “in phase”- basically meaning that they have the same value at the same time (sort of, but not really like a delay difference of 0) – the result is a sine wave that is in phase with the other two with double the amplitude of the two inputs.
Now, let’s move one of the two signals in time. We’ll make it late by half the length of the sine wave (in time) and see what happens.
Now you can see that, since the bottom plot is the negative of the top plot at any given moment in time (because we’ve delayed it by half a “wave”), when you add them together, you get no output.
Let’s look at one last example, where the two input signals have some phase difference that is not quite so simple. This is shown in the figure below.
Again, you can see that, when you add two sine waves of the same frequency, you get another sine wave of the same frequency. You can also see that the phase (remember – phase is something like delay) of the result is not the same as the phase of either of the two inputs. Finally, you can see that the amplitude (the maximum value) of the result is neither 0 nor 2 – it’s something in between.
So, the moral of this story is that, if you have two sine waves then the result (what you hear) is (at least partly) dependent on how the two signals add – and that result might mean that you get something twice as loud as either input – or it could mean that you get nothing – or you get something in between.
The real world
The three plots shown above illustrate some simple examples of what happens when you have two sound sources that are added together to produce a result. For the purposes of this article, the two sources are two loudspeakers – the “main” loudspeaker and the subwoofer, and the result is the sum of those two signals at your listening position. Let’s go back to thinking about what frequency ranges are produced by these two loudspeakers. The plot below shows the magnitude responses of the filters in a BeoVision 11’s internal bass management system at its default crossover frequency of 120 Hz. The red curve shows the response of the low pass filter whose output is sent to the subwoofer output. The black curve shows the response of the high pass filter whose output is sent to a main loudspeaker.
Take a look at the response of the signal that is processed by the low pass filter. You’ll notice that, although the “crossover frequency” is set to 120 Hz, there is still signal coming out of the filter (and therefore out of the subwoofer) above that frequency – it’s just getting quieter as you go further up and away from the crossover.
You might also notice that, at the crossover frequency, the level of the signal coming out of the low pass filter is identical to the level of the signal coming out of the high pass signal. That’s (more or less) what makes it the crossover frequency. As you move down from that frequency, you gradually get more out of the low pass (the sub) than the high pass (the main). As you move up in frequency, the opposite happens. However, there is a region around the crossover where both loudspeakers are contributing roughly equally (within reason) to the signal that you get at the listening position. So, the “truth” is a little more like the plot below:
I’ve chosen -20 dB as the point where I can start ignoring a signal, but that’s a pretty arbitrary decision on my part. I could have set my threshold higher or lower and still argued that I was right. So, if you disagree with my choice of -20 dB as the threshold of “I don’t care any more” then I agree with you. :-)
By now, you should start to worry a little. You should be asking something like “hmmmm… you’re telling me that there is a big band of frequencies (say, roughly between 1 and 2 octaves) right around where human voice fundamental frequencies sit (well, at least my voice sits there – but I sing bass…) where a bass management system will send the signal out of two loudspeakers!? AND, to add insult to injury, you told me (in the previous section) that if the phases and amplitudes of those signals from those loudspeakers aren’t perfectly aligned, the result at the listening position won’t be the same as the input of the whole system?” If you ARE asking something like that, then you’re in good shape. As has been said by many other people in the past: the first step in fixing a problem is admitting you have one.
So, let’s ask a different question: what parts of the audio signal chain could affect either the amplitude or the phase of the signals coming out of the loudspeakers? Brace yourself… This list includes, but is not exclusive to:
- The characteristics of the filters in the bass management system
- The characteristics of the filtering in the loudspeakers
- The physical principal of the loudspeaker (i.e. sealed cabinets will be different from ported loudspeakers which are different from passive radiators)
- Diffraction (although this might be a small issue)
- Latencies (total delay) of the loudspeakers (for example, digital loudspeakers have a bigger delay than analogue ones typically)
- Distances of the loudspeakers to the listening position
- The characteristics of the room itself
- And more!
All of these issues (including the ones that fall under the “And more” category) have some effect on the phase (and amplitude) of the signal that you hear at the listening position. And, since some of these (like the distances and the room characteristics) are impossible for us (as a manufacturer) to predict, we have to give you, the end user, some way of adjusting your signals so that you can compensate for misbehaviour in your final system.
Now, although this is a “technical” article, I think that it would be too technical to start looking at the specifics of the phase responses of sealed cabinet vs ported loudspeakers, for example, since the details will be messed up by the listening room anyway. So, instead of getting into too many details, let’s just say that “you can’t expect your system to work perfectly without tweaking it” (see the reasons above) and just talk about some strategies for setting up your system so that it behaves as well as it can (without going out and hiring an acoustical consultant).
BeoLab 19 Controls
The BeoLab 19 has a number of controls that have not been available on previous B&O subwoofers. As a result they may cause a little confusion and playing with them without knowing what to expect or listen for could result in your system not performing as well as it could. On the other hand, it could be that these controls could help you improve your system if you’re finding that it’s not really behaving. Let’s take each control, one by one, and explain what it does, and talk about strategy afterwards.
Gain
The gain knob (on the left in the diagram above) is basically just a volume knob that controls how loud the subwoofer is overall. Let’s say, for example, that you have a perfectly configured system, then the outputs of the subwoofer and the main loudspeaker mate perfectly and result in a perfectly flat response below, through and above the crossover region. (this never happens in real life – but we can pretend). The diagram below shows an example of this, where the top plot shows the outputs of the subwoofer and main loudspeaker and the bottom plot shows the total result at the listening position.
If you do nothing but change the gain of the subwoofer, (using the Gain knob on the BeoLab 19, for example) then the result would be something like the plot below.
You can see in the plot above that all you do is to boost a region of low frequencies without doing anything strange through the crossover region. So, if you like bass, this might be a nice tweak for you. However, in theory, your goal is to get a response like the one in the first plot, where the outputs of the subwoofer and main loudspeakers have the same level (at the listening position).
LP Filter
One possible configuration of the BeoLab 19 is to connect it in parallel with your main loudspeakers and to not use and external bass management system.
If you do this, then you are relying on the fact that the main loudspeakers have a high pass filter built-in, and you will align the low pass filter inside the BeoLab 19 to have approximately the same frequency so that the total result is a smooth-ish crossover region. In order for the low pass filter to work, you will have to turn it ON using the switch. (Note that, if you’re using an external bass management system as in the BeoVision 11, for example, then you should turn the low pass filter OFF, thus removing it from the signal path of the subwoofer.)
In theory, the goal here is to match the cutoff frequencies so the two loudspeakers behave nicely together across the crossover region. For example, if the natural low frequency cutoff is about 50 Hz, and you set the LPF in the subwoofer to 50 Hz, then you get the result below
What would happen if you set the LPF incorrectly – let’s say that you make it higher than the correct value, since you would think that, by overlapping the sub with the main speaker, you’ll get more output and impress the neighbours. Well, the result would be the plot below.
As you can see, although the sub is now delivering more signal (because it’s going all the way up to 120 Hz instead of 50 Hz in the previous plot), you actually get a reduction in the total output of the system. This may be initially counterintuitive, but it’s true in our example, since (as you may remember from something I said earlier in this article) the phase of the subwoofer is, in part, determined by the characteristics of the filtering in the loudspeaker. By changing the low pass filter frequency, we change the phase of the subwoofer in the crossover region and result in a cancellation with the main loudspeaker instead of a summing. In essence, both the sub and the main loudspeaker are now working very hard to cancel each other (especially around 80 Hz or so) and you hear very little at the listening position.
On the other hand, I have assumed here that the main loudspeaker’s high pass filter is a very specific type. A different main loudspeaker with a low frequency cutoff of 50 Hz would have had a completely different behaviour as you can see below.
So, the moral of the story here is that setting the low pass filter frequency will have some effect on your total response. However, you should not jump to the conclusion that you can predict what the frequency should be – you will have to fiddle with the knob whilst listening to or measuring the total output of the system. You should also not jump to the conclusion that increasing the frequency range that is covered by the subwoofer in a parallel configuration will result in more output from your system. Overlap is not necessarily a good thing – sometimes, more is less…
Phase
Go back up and take a look at the two sine wave in the plots in Figure 4. One way to describe these two waves is to say that the middle one is half a wave later than the upper one – in other words, they are 180º out of phase. Another way to describe them is to say that the middle one is the inverse of the upper one – they have the same instantaneous value at any time, except that they are the negative of each other (in other words, signal 2 = signal 1 * -1).
So, intuitively, you can see that shifting the phase of a signal by 180º is the same as flipping it upside down. This could mean that, for example, all other things being ignored, that when a kick drum tells your subwoofer to push outwards, shifting the phase by 180º will result in your subwoofer sucking inwards instead. However, this is only true if all other things are being ignored. As soon as your subwoofer has a high pass filter (i.e. a low frequency limit) and a low pass filter (a high frequency limit) and it’s a loudspeaker driver in a cabinet in a room, all bets are off. All of those aspects (and more!) will have some effect on the phase of the system, so you can’t predict whether the kick drum will cause the woofer to put out or suck inwards.
So, instead of worrying about the “absolute phase” of the subwoofer, it’s more interesting to worry, once again, how it matches up with the main loudspeaker. Let’s take exactly the same responses from the plots shown in Figure 14 above (which didn’t add together so well for some reason) and shift the phase of the sub by 180º using the Phase switch. The result is shown below in Figure 15.
As you can see, the big dip in the total response of the system (seen in Figure 14) has been corrected, and we now have more output (actually, a little too much) below that. So, the result is that the total system response is much better than it was without flipping the phase switch.
Of course, if we flipped the phase switch in a system that was behaving nicely, then bad things might happen. Let’s flip the phase on the system shown in Figure 9, for example. That total result would look like the one in Figure 16, below.
As you can see, you get the same amount of low bass in Figures 9 and 16. However, there is a nasty dip at the crossover frequency of 120 Hz when the two loudspeakers are cancelling each other.
So, the moral of the story here is that, if you have a problem in the crossover region between the main loudspeaker and the subwoofer, flipping the phase of one of the two might help the situation – although it might make things worse…
Pos (aka Position)
Almost every loudspeaker in the Bang & Olufsen portfolio has a switch that lets you change the characteristics of the loudspeaker to compensate for the differences in its response as a result of its placement in a room. Generally speaking, the closer you put a loudspeaker to a wall, the more bass you’ll get out of it. If you put it closer to two walls (i.e. in a corner) you’ll get even more bass. However, that is a very general characterisation – the reality is that you’ll get a little more at some frequencies and a little less in other frequencies – and that behaviour is dependent on the diameters of the loudspeaker drivers, the crossover frequencies, and the physical shape of the loudspeaker.
So, without getting into the details of exactly what is being changed in a BeoLab 19 (or BeoLab 2 or BeoLab 11 – or any other loudspeaker for that matter), let’s say that you should put the position switch in whatever setting best corresponds to the location of the loudspeaker in your room. However, if you want, you could cheat a little and fiddle with the switch to see if you like another setting more.
For example, if your loudspeaker is in the corner, and you put it in “free” mode, you’ll get LOTS of bass – too much bass. But if you like bass, this is one way to get it. Of course, there are other implications to this decision, but if you like bass enough, that might be reason enough to change to the “incorrect” the switch setting.
Wired / Wireless
The BeoLab 19 has the ability to receive its input via the analogue or digital input OR via the wireless receiver module that is built into it. This switch merely tells the loudspeaker whether it should “listen” to the wired input (either analogue or digital) or the wireless one. (Note that the BeoLab 2 and the BeoLab 11 do not have wireless receivers.)
L / R/ L+R
Most subwoofers (including the BeoLab 2 and the BeoLab 11) are built with the assumption that you will use them either:
- as a stand-alone subwoofer in a multichannel (i.e. 5.1 or 7.1) system where it gets the “.1” output from the source (that may, or may not have bass management) and so you just send one audio channel into it OR
- in a 2.1 setup where you want the left and right channels coming into the subwoofer where they are added together produce a mono bass signal internally
Consequently, most subwoofers either have 1 input (assuming that they are to be connected to the “subwoofer out” on something like an AVR) or a 2-channel stereo input (assuming that they should “see” left and right) that is summed to mono. BeoLab 2 and 11 are built based on the second assumption.
BeoLab 19 allows you to use the subwoofer in either of these configurations. So, in either “L” or “R” mode, it is only “listening to” the Left or Right audio channel on the Power Link input. In “L+R” mode, the input of the subwoofer is taking both audio input channels and summing them to make a mono input to the subwoofer. Note that, if you send exactly the same signal on the Left and Right audio channels on the Power Link cable, and then you switch the BeoLab 19 from either L or R to L+R, you’ll find that you get a doubling in the output level. This is because a signal plus itself is twice as loud. Since this is what BeoLab 2 and 11 do all the time, if you simply replace a BeoLab 2 or 11 with a BeoLab 19, you should put the 19 in “L+R” mode – otherwise you’ll lose some bass in your system.
However, if you want to use a single Power Link cable to run to the Subwoofer and to another loudspeaker (say, a centre channel, for example), then you should put the BeoLab 19 in either L or R mode (and the other loudspeaker in the opposite mode) so that you can access both loudspeakers independently. This is also the case if you want to run two BeoLab 19’s on the same Power Link cable and use the 2-channel LFE output option in a BeoVision 11. In this case, you se one BeoLab 19 to “L”, the other to “R” and set the Speaker Roles in the BeoVision 11 to “Sub Left” and “Sub Right” (or “Sub Front” and “Sub Back”) appropriately.
Note that, if you are in Wireless mode, the “L/R/L+R” switch does nothing.
How to do it (Finally!)
Method for an Externally Bass Managed Configuration
If your main loudspeakers and your subwoofer are connected to a system that has a bass management system, then you should use it. There are a number of reasons for this:
- the main loudspeakers may behave better (for example, with respect to distortion or port noise) if they are not being pushed by a lot of bass
- a bass management system will work for a multichannel loudspeaker system
- a bass management system (for example, in a BeoVision 11) will be capable of making some “intelligent” decisions with respect to your entire system
- a good bass management system (for example, in a BeoVision 11) will allow you to make fine adjustments to accommodate your configuration and room
So, your procedure here (assuming that you have a BeoVision 11 and a BeoLab 19 and some main loudspeakers) is as follows:
- Turn off the LP Filter on the BeoLab 19, set the Phase to 0, set the Gain to 0, and set the other switches to whatever is best for your particular configuration.
- Put the correct loudspeaker models into the Speaker Connections menu on the BV11.
This will compensate for differences in the latencies and sensitivities of the loudspeakers, in addition to making some intelligent decisions about where to route the bass. - Set your Speaker Distances correctly
This will ensure that you do not have phase differences in the loudspeakers at the listening position as a result of problems caused by the speed of sound and mis-matched distances. - Set your Speaker Levels correctly
On a BeoVision 11, this is done by making sure that, at the same volume level, all loudspeakers produce the same level in “dB SPL, C-weighted, Slow” on an SPL meter like this one, or this one, or this one, for example. - Turn on a piece of music that has a constant bass level
The opening of Freddy Mercury’s “Living on my Own” or Santanta’s “You Are My Kind” are a possible tunes. Claire Martin singing “Black Coffee” is also a good candidate. If you want to look like a professional, then I suppose that you could use pink noise or this track instead of music. - Sit in the listening position and listen to the total behaviour of the system. Pay particular attention to “unevenness in the bass”. In other words, listen to the bass and pay attention to whether some notes are quieter or louder than others.
- In theory, if you performed Step 4 correctly, then you shouldn’t have to play with the Speaker Level in the TV or the Gain on the subwoofer.
- If there is a general area somewhere in the middle of the bass where lots of notes are too quiet, try flipping the phase on the subwoofer.
- If some individual frequencies (or notes) are quiet then playing with the allpass filter on the TV might help.
- If some individual frequencies (or notes) are louder than others, this is probably caused by the room, and you might be able to deal with it by moving the subwoofer. If, when you put the sub in the corner, you make this problem worse, it is almost certainly the room acoustics that you’re dealing with, so moving the sub is your best bet.
Method for a Parallel Connection Configuration
- Turn the LPF frequency as low as you can go
- Turn on a piece of music that has a constant bass level
- The opening of Freddy Mercury’s “Living on my Own” or Santanta’s “You Are My Kind” are a possible tunes. Claire Martin singing “Black Coffee” is also a good candidate. If you want to look like a professional, then I suppose that you could use pink noise or this track instead of music.
- Sit in the listening position and ask someone to turn the LPF as low as it will go.
You should notice that there is a “hole” in the level of the bass between the subwoofer and the main loudspeaker. Turn up the LPF frequency and pay attention whether the “hole” fills up or gets worse. If it gets worse, flip the phase switch and start Step 4 again. - If the hole did not get worse, then keep turning up the LPF frequency until it sounds like there the hole is filled up.
- One you’re done playing with the LPF frequency, try moving the Gain to adjust the bass to the level that you like.
Addendum
There are some more examples of what happens when you play with the various knobs on a subwoofer here.
Loudspeakers are not just for making noises…
B&O Tech: What is Sound Design?
#7 in a series of articles about the technology behind Bang & Olufsen loudspeakers
My official job title at Bang & Olufsen is “Tonmeister and Technology Specialist in Sound Design”. The second half of that title is a bit weird – what is a “sound designer” and why would B&O want to have one on staff?
To answer that question, let’s start by talking about how a loudspeaker behaves in a real room. In many respects, a loudspeaker is like a lamp. Turn on a lamp and look at where the light goes. Some of it goes directly on what you want to look at – like the book you’re trying to read, for example. More of the light goes in other directions – it radiates outwards and reflects off of the walls, floor, ceiling, and furniture. And, if your lamp is anything like the one in my living room, then the light that shines directly on your book is not the most important part. In fact, if there was no direct light shining on the book, you would probably still be able to read your book because of the light reflecting towards your book off of everything else in the room.
A loudspeaker is basically the same thing – you have some sound that radiates directly out of the loudspeaker towards the listening position (assuming that the loudspeaker is “aimed” at the listening position) like a laser beam (like the light shining directly on your book – this is called the loudspeaker’s “on-axis magnitude response” or the “frequency response“). In addition to this, you have the sound that radiates outwards in all directions simultaneously like a big ball expanding in all three dimensions (this is called the “power response” of the loudspeaker). There are a couple of things to think about here. The first is that the sound that is coming directly from the loudspeaker to the listening position isn’t necessarily in the direction that the people who built the loudspeaker would call “on-axis” – in fact, more than likely, it’s slightly off-axis. This is okay, since you can think of a loudspeaker’s principal axis of radiation more like the beam from a flashlight than a laser beam (so you have a “listening window” instead of a single spot). The second thing to remember is that, in most listening situations, you are hearing FAR more energy from the loudspeaker’s total power response than you do from the direct sound’s magnitude response. In fact, in a lot of situations, you don’t have any direct sound at all – just power response filling up the room and reflected back to you. (If you’d like to learn more about this concept, read this posting to start off.)
The (rather important) moral of this short story is that the power response is at least as important as the magnitude response – and usually much more important. The problem is that, if you read loudspeaker reviews in magazines, you get the impression that the on-axis magnitude response is the most important thing there is to know about a loudspeaker. This is simply not true – the on-axis response of a loudspeaker is one of the easier things to measure, so that’s what gets measured by most people. It’s very difficult to do a reliable power response measurement, so most people don’t do one. Keep this in mind as you keep reading.
So, what are the steps we take when we tune our loudspeakers during the development process?
Step #1: Measurements
The prototype is put on the crane in the Cube and the linear part of its acoustic response is measured by the acoustical engineer assigned to the project. The output from these measurements consists of four final measurements:
- the on-axis frequency response
- the frequency response of the loudspeaker at a lot of different angles in all directions (not just around the loudspeaker’s “equator” but also above and below it)
- a kind of an average response in a “listening window”
- the power response (made by adding the results of all the measurements done in all directions)
Since we make DSP-based active loudspeakers (unlike passive loudspeakers) the angular direction that is chosen as the “on-axis” location is arbitrary. This is because the final response from each loudspeaker driver and the delays that are used to time-align them are determined by the filtering that we apply in the DSP. I’ll talk about this in a future posting. However, what this means is that “on-axis” is “wherever we decide it to be” – NOT “directly in front of the tweeter” or “directly in front of the loudspeaker”. So, we do a measurement of the frequency response (which is comprised of the magnitude response and the phase response) of the loudspeaker in the absence of any wall reflections at some distance, on a line that has been determined to be the “on-axis” direction.
The power response of a loudspeaker is kind-of-sort-of the sum of its magnitude responses in all directions. This is essentially a measure of the total acoustic energy that a loudspeaker sends into a room in all directions at the same time. So, instead of thinking of a loudspeaker as a laser beam (as in the on-axis response), this considers the loudspeaker as a naked light bulb, sending sound everywhere (which is actually a little closer to the truth).
The listening window is an area that has the on-axis line as its centre. It’s an oval-shaped area that is wider than it is high, that represents an area in front of the loudspeaker where we think that this listeners will typically be located.
In the old days (actually, for all of the active loudspeakers before BeoSound 8), the result of this process would have been two filters. The first would be a correction filter made by the acoustical engineer that made the loudspeaker’s on-axis frequency response flat in its magnitude response. The second would have made the power response smooth (i.e. without too many dips and bumps). Then the loudspeaker would have gone into the listening room, with those two filters as two different options as starting points for the listening-based sound tuning.
Nowadays, we do things a little differently, the measurements that are performed in the listening window (between 10 and 20 measurements in total) are compared and analysed for common aspects in their time responses. In other words, we’re checking to see whether the loudspeaker has natural resonances in it that causes it to “ring” in time (just like a bell rings when you hit it). Ringing is a natural behaviour of a loudspeaker, but that doesn’t mean it’s a good thing – it means that some frequency (the one that’s ringing) lasts longer than the others when you hit the loudspeaker with a signal (usually it’s ringing at lots of different frequencies). Depending on what frequencies are ringing, the result could be a “muddy”- or a “harsh”-sounding loudspeaker (to name just two of many descriptors meaning “bad”…) If we can see the same ringing in all (or at least most) of the measurements in the listening window, then the DSP engineer working on the project will make a filter for the signal processing that makes the ringing go away – in essence, we make the signal that we’re sending into the loudspeaker ring opposite to the natural ringing of the loudspeaker itself. You can think of it like kicking your legs in the wrong direction when you’re on a swing to make yourself slow down – you’re actively working in the opposite direction of the natural resonance of the system (where you-on-the-swing is “the system”).
In addition to making the resonances go away, we add filters to
- push the low frequency response to go as low as we want it to
- ensure that the loudspeaker drivers (for example, the woofer and the tweeter) meet each other correctly through the crossover and work together instead of against each other. In order to do this correctly, you can’t just build a crossover – you have to incorporate the natural frequency responses of the loudspeaker drivers as part of the total filter design.
At the end of this process, we have a loudspeaker that has a final response that has been corrected so that it measures well inside the listening window. We also have a bunch of measurements that we’ll probably come back to later.
Step #2: Tuning
The prototype with its correction filters are brought into the listening room and we start playing music through it. The first thing to do is just sit and listen using recordings that we know really well (usually, for me, that means starting with “Bird on a Wire” by Jennifer Warnes from Famous Blue Raincoat – I’d guess that I have heard that song, on average, once a day, every day, since about 1990 or so). Pretty soon, some problem will be apparent. Depending on the problem that shows up, we’ll try to fix it by correcting the physical reason for the problem. (This is done by the acoustical engineer working with the mechanical engineers to sort out where the problem occurs and how to fix it.) For example, if a part of the loudspeaker cabinet is vibrating and “singing along” with the loudspeaker, we’ll stiffen the cabinet, either by increasing its thickness, or changing the material it’s made out of, or adding ribs or bulkheads or some combination of those things. Once that problem is fixed, we bring it back into the listening room, find another problem, fix it, listen, complain, fix, listen, complain, fix, rinse, repeat, etc. etc…
Eventually, once all of the problems that we can fix with physical corrections are done, we start the next phase of the tuning. This is where the “design” part of the sound design comes in…
We set up the loudspeaker with its correction filters and its physical improvements in the listening room and start listening to music again. Now, if something sticks out as sounding wrong in the recording, we use an equaliser to correct it. If a note is sticking out that shouldn’t be, then we put in a dip in the equalisation to reduce the problem. If there’s some frequency band that seems to be missing, then we’ll use an equaliser to boost it a little to get it back. Typically, that process takes about 3 to 5 days in the listening room, and at the end, we have something between 20 and 40 extra equalisers in the signal flow. When that’s done, we pack up and go to a different listening room and start the process from scratch again. A couple of days later, we have another 20 – 40 filters. Then we pack up and go to a different room and start again. That process is done in something like 4 or 5 rooms, depending on the loudspeaker that we’re working on. Usually, we try to use rooms that are different from reach other, but also that will be representative of the acoustic behaviours of the rooms that the products will be used in. For example, when we were tuning the BeoPlay A9, one of the “rooms” was outdoors in the acoustical engineer’s back yard. This was because some customers will put their A9 out on the back deck or by the pool – so we used that situation as one of our tuning rooms.
So, now we have 4 or 5 sets of tunings, each with about 30 equalisers in them (give or take…). These tunings are then analysed to see what is common amongst them. You see, if we were to just tune a loudspeaker in a single position in a single room, a big part of the tuning would be there to correct the acoustical behaviour of the room. For example, in our main listening room in Struer, we have a pretty nasty room mode that rings at about 55 Hz. Whenever I tune a loudspeaker in that room, I put in a notch filter at 55 Hz to reduce the audibility of the problem (especially since I start tuning using Bird on a Wire, and it’s in A Major, and 55 Hz is an A). However, if your living room is not the same size as Listening Room 1 in Struer, then your room modes will be at different frequencies, so you should have a notch filter at those frequencies instead of 55 Hz. So, in order to eliminate the individual corrections for the individual rooms that we used for tuning the loudspeaker, we just take the common aspects from each tuning. For example, if the first tuning has a dip at 55 Hz and a boost at 200 Hz, and the second tuning has a dip at 65 Hz and a boost at 200 Hz – we only keep the 200 Hz boost (since the notches at 55 Hz and 65 Hz are probably due to the rooms, not the loudspeaker itself).
Once the common aspects of all those tunings have been extracted, we use those to build an equalisation that is, essentially, the sound design. That equalisation is built into the loudspeaker, and we start getting more people to listen to it in more rooms (for example, we’ll send people home with prototypes that include the sound design tuning to get “real world” testing).
Why do you need Sound Design?
Of course, there are purists amongst you who will ask why it is that we need the sound design process in the first place. The logic goes that if you make a loudspeaker with a razor-flat on-axis frequency response, then you will get a perfect loudspeaker – end of story. Anything that is done afterwards to muck about with that response is just ruining the loudspeaker. Ignoring a lot of details, this would be true if you used your loudspeaker in a room that had no reflections – in other words, if all you hear is the on-axis sound, and all of that energy that goes in all other directions never reflects off of anything else and bounces back at you, then a flat on-axis response would probably be a good idea.
However, think back to where we started. We said that the power response of the loudspeaker is at least as, if not more, important than the on-axis magnitude response. This means that the sound that radiates away from the loudspeaker in directions other than yours is what you hear most of the time. The relationship between the on-axis magnitude response and the power response is determined by the physical shape of the loudspeaker and its components (as well as the frequencies of the crossovers). And, how that balance between the on-axis response and the power response is perceived at the listening position (wherever that might be…) is really unpredictable. So, rather than building a tuning that is based on a prediction, we experience it instead – by playing the loudspeaker in different rooms and different positions and assembling some sort of average behaviour in the real world.
One of the statements I’ve made on Bang & Olufsen marketing materials in the past (like this video, for example) is that, when you sit in your living room and listen to a pair of B&O loudspeakers, you should hear what the mastering engineer (or the mixing engineer, or the recording engineer) heard when he or she did the recording using professional studio monitor loudspeakers in a mastering or recording studio. (Note that this is very different from the philosophy that you should be able to sit in your living room, close your eyes, and be fooled into thinking that the musicians are standing in front of you. In my opinion this is a silly philosophy, akin to believing that you should go to a movie theatre and believe that you’re in the movie instead of watching it. A music recording should be better than real life – not the same as it. And, please – before you write a comment below telling me that I’m wrong, read this first – then this – and then come back and write a comment below telling me that I’m wrong.) However, since your living room is not a mastering studio, it doesn’t make sense for you to use studio monitors. In other words, the goal is that the combination of B&O loudspeakers and your living room should be the same as studio monitors and a recording studio.
So, the moral of the story is that the goal of sound design (at least at Bang & Olufsen…) is to ensure that our loudspeakers in a normal room (whatever that means for a given product) sounds like professional studio monitors in a recording studio. In other words, if we started making studio monitors instead of home loudspeakers, I’d be out of a job, since we wouldn’t need a sound design procedure to “undo” the effect the room has on our loudspeakers…
P.S
One thing that I did not talk about here (mostly just to keep things clear) was the off-axis responses of the loudspeaker, the collection of which comprises its directivity. That discussion will be left for a future posting.
P.P.S.
There is one aspect of this article that can explain one issue that some people have with B&O loudspeakers. If you take a look at some magazine reviews and some comments from people-who-post-opinions-about-loudspeakers-late-at-night-on-Internet-fora, they’ll say that our loudspeakers are obviously not worth anything, since they do not have a flat on-axis frequency response. Of course, if the only criterion you use to define what makes a loudspeaker “good” is a one-dimensional measurement at a single point in space, then you might be inclined to agree with that opinion.
However, if, like me, you live in three dimensional space in a house that has walls, floors and ceilings – and you have more than one chair and possibly even a friend or two – you might be inclined to think differently…
B&O Tech: What are subwoofers REALLY for?
#6 in a series of articles about the technology behind Bang & Olufsen loudspeakers
The Setup
Back in a previous posting, I said something that could be perceived as interesting… The short version of what I said there was that, if you’re making a DSP-based active loudspeaker (like all of the new loudspeakers in the B&O portfolio), you can essentially make it sound like whatever you want. You do this by adding filters in the digital signal processing (DSP). (Let’s assume for this article that we’re only talking about the on-axis magnitude response of the loudspeaker, and we’re working in an anechoic environment (aka a “free field” situation), since that will keep things simple.) This means that, if I can apply enough boosts and cuts, I can get any magnitude response I want out of the loudspeaker. In other words, I can have a 1″ tweeter that plays with a perfectly flat response from 20 Hz to 20 kHz.
However, there are some serious restrictions on this statement. As a minor example, if there is a problem with diffraction the only way to change that is to modify the shape of the loudspeaker cabinet (if you don’t know what diffraction is, don’t worry – it will not be mentioned again in this article).
However, there is one GIANT restriction on the statement that we’ll look at this week. This is a question of how loudly you want to play. So let’s look at that.
In order to make sound, a loudspeaker driver has to move in and out – this pushes and pulls the air molecules in front of it, creating small areas of higher pressure and lower pressure (relative to today’s natural barometric pressure) that radiate outwards, away from the driver. Those variations in pressure push and pull your eardrum in and out of your head which, in turn cause stuff to happen in your inner ear which, in turn causes stuff to happen in your brain – but that is all outside the scope of this discussion.
Back to the loudspeaker – it has to move in and out. The louder you want to play (more accurately, the higher the Sound Pressure Level (SPL), the more it has to move in and out. Also, the lower in frequency you want to play, he more it has to move in and out (to keep the same SPL).
The real problem is the second of these, since the rule of thumb is that, every time you go down one octave (in other words, you divide the frequency by 2) you need to quadruple the excursion of the driver (the amount it moves in and out).
Let’s look at an example. The figure below illustrates the excursion required for different sizes of loudspeaker drivers in order to create a sound pressure level of 60 dB SPL (which is not very loud – but is a typical sort of listening level) at 1 m (which is a good approximation for how loud it will be all over your living room due to something called the room’s “critical distance” – we’ll talk about that in the future).
Notice that, for the 15″ woofer, it only has to move 0.08 mm out of the box (and 0.08 mm into the box) to produce a 20 Hz signal at 60 dB SPL. This is not very much movement. By comparison, the 4″ woofer has to move 1.2 mm which is much more than 0.08 mm, but still not much.
To bring this into the real world, this means that a woofer taken out of a BeoLab 3 (which is 4″ in diameter) would have to move 14 times farther than a woofer from a BeoLab 5 (15″ woofer) to produce the same output. This is because the 4″ woofer is smaller than the 15″, so to move the same number of air molecules, we have to move it more. (actually, what we’re really thinking about here is how many litres of air we’re moving, but that might be too much detail…)
Let’s consider the practical implications of this graph. Since a BeoLab 3 woofer can move 1.2 mm in and out (and, of course, a BeoLab 5 woofer can move 0.08 mm). Both loudspeakers are able to produce a 20 Hz tone at 60 dB SPL. Therefore, if we choose to do so, we can make both loudspeakers have a magnitude response that was flat from 20 Hz to 20 kHz at this listening level (or quieter).
Let’s turn up the volume knob. We’ll go up to 80 dB SPL which is a bit loud, but certainly not enough to get the party going… Now we need to move the 15″ woofer 0.8 mm (still not very much…) and the 4″ woofer 11.6 mm to produce 20 Hz at 80 dB SPL. Of course, the BeoLab 5 woofer can easily move 0.8 mm, but 11.6 mm is too far to go for the BeoLab 3 woofer. So, if we didn’t have ABL to protect things from moving too far, we would not be able to tune the BeoLab 3 to be flat down to 20 Hz – we would have to “roll off” the low frequencies so that 20 Hz was not as loud as the frequencies above 20 Hz in order to prevent it from causing the woofer to move to far when you turn up the volume. (For example, we could tune it to be flat down to 40 Hz instead of all the way to 20 Hz.)
Let’s go further, just to make things really obvious. We’ll turn up the volume to 110 dB SPL (which is very loud). Now, to get a 20 Hz tone out at this level, the 15″ driver will have to move 2.6 cm and the BeoLab 3 woofer would have to move 36.6 cm (which is silly). So, here it is obvious that, if we want to build the BeoLab 3 to play 110 dB SPL, we will have to use ABL or limit its low frequency content (or use some balance of those two things – a little ABL and a little higher low-frequency limit).
Let’s look at this in another, more intuitive way. If we wanted a BeoLab 3 woofer to play as loudly as a BeoLab 5 woofer can play, at its peak excursion in and out of the cabinet, it would look like the figure below.
The Implications
So, what does this mean? Well, it means two things:
- for normal listening levels, we can use our DSP to make our loudspeakers have as much bass as we choose
- however, this means that we need ABL to reduce the bass at higher listening levels
But, what happens if you want to buy BeoLab 3’s (or another “small” loudspeaker in the B&O portfolio), but you don’t want to lose bass output at high listening levels? Well, you have two choices:
- buy bigger loudspeakers
- buy a “subwoofer”
“What’s a subwoofer?” I hear you cry. Well, let’s be honest to start. In theory, a subwoofer is a loudspeaker that should play frequencies that are below the limits of the woofer. (In a system with passive loudspeakers, this would actually be true.) However, in a DSP-based, fully-active loudspeaker system, a subwoofer has a slightly different role. In the case of a Bang & Olufsen system, a subwoofer behaves more like a woofer with more ability to play loudly than the main loudspeakers.
For example, if you have a pair of small loudspeakers (let’s say, the built-in loudspeakers in a BeoVision 11, for example) and you add an external subwoofer (say, a BeoLab 19), and you’re listening at normal listening levels, then (all other things being equal) turning the subwoofer on and off should not produce a noticeable change in the bass level. In fact, if you turn on the subwoofer and hear a difference, it means that the subwoofer is too loud.
However, if you turn up the volume, you will get to a point where the “small” loudspeakers cannot produce enough output at low frequencies, so the ABL starts turning down the bass to protect the loudspeakers from distorting. Now, since the subwoofer can play louder at low frequencies, you will notice the difference.
Of course, this assumes that you’re using something called “bass management” which is an algorithm that removes the bass from the signals sent to your small loudspeakers and re-directs it to the more capable subwoofer. So, in the example above, where I was suggesting that you were turning your subwoofer on and off, I should have been more specific, since turning your subwoofer on implies that you’ve removed bass from the small loudspeakers at the same time.
This has a secondary implication. This means that, if you have a two different types of main loudspeakers (i.e. BeoLab 5 in as your front Left / Right pair and BeoLab 12 and your surround Left / Right pair) then we can do the same “trick”. So, the bass management system should “know” that the 5’s have more capability to play low frequencies louder than the 12’s and automatically direct the bass from the surround channels to the BeoLab 5’s in the front (therefore making the BeoLab 5’s the front loudspeakers and the subwoofers). And, if we were REALLY smart, the “brain” at the centre of the system would know the bass capabilities of all loudspeakers that are attached to it and be able to make intelligent decisions about who should get the bass. This is exactly what is happening in the BeoVision 11, BeoPlay V1 and BeoSystem 4. When you enter your Speaker Types (the model numbers of the loudspeakers in your configuration), the software inside the television automatically decides whether the bass should be redirected from a given loudspeaker in the configuration to another loudspeaker, based on the maximum outputs of those loudspeakers at low frequencies. (This entire lookup table is shown in the Technical Sound Guide available here – a small section of the table is shown below.)
There is one small thing that I haven’t mentioned, but some sticklers-for-detail will want that I do so… The reason you can get away with doing this whole bass-redirection-trick is that, in a normal listening room, we humans are worse at localising where low frequencies are coming from than we are for higher frequencies. This inability on our part can therefore be exploited by moving the bass to a different loudspeaker. However, there are some people who say that this inability is over-estimated (in other words, some people say that we’re better at locating subwoofers than most people think we are) however, that debate can probably be addressed by discussing the size of the room and how low a frequency is “low” – and those are just excruciating minutiae (at least, within the limits of this article…)
B&O Tech: TrueImage Upmixing
#5 in a series of articles about the technology behind Bang & Olufsen loudspeakers
The the BeoSystem 4, the BeoVision 11, and the BeoPlay V1 all use Bang & Olufsen’s “TrueImage” algorithm – but what is TrueImage and why did we choose to use it instead of a commercial standard from someone else?
The Source: How many channels are coming in?
The first question we have to address is that of how many channels your source has. There are many standards these days for audio – particularly if we’re talking about audio that accompanies video.
1 channel – mono: If you’re watching the evening news, you’re probably getting a single audio channel which is basically a feed from the new anchor’s microphone. This might also be the case if you’re listening to a podcast – maybe…
2 channels – stereo: Technically, “stereo” doesn’t automatically imply only two channels – although most people mean “two channels” when they say “stereo”. (I am equally guilty of this behaviour.) There is a plethora of materials recorded in 2-channel stereo, going as far back as the 1940’s! Lots of television channels are broadcast in 2 channels (I remember a time when, if you wanted to hear a TV broadcast in stereo, you had to tune your FM stereo for the audio being simultaneously broadcast on the radio…). Almost all music is distributed in 2-channel stereo. Actually – almost everything except for movies and video games has two channels of audio. In case you aren’t already aware,
- there are two channels because you have two ears – one channel is for the left, and the other is for the right
- stereo was originally patented by Alan Blumlein in the 1930’s
- Blumlein originally patented stereo for sound “especially when associated with picture effects as in talking motion pictures” – it wasn’t originally for sitting and just listening to music – no snobbery!
- Just because there are two channels of audio doesn’t necessarily mean that those two channels are different. As I type this, my two kids are watching a television show that is in mono, but broadcast as a 2-channel signal. All that means is that the two audio channels are identical. Most people call this “dual mono”.
4 channels: For a while, there was a system available from Dolby called “Dolby Stereo” in cinemas, but “Dolby Surround” in home systems. These two were essentially the same thing. This worked by encoding (sort of…) 4 channels of audio (Left Front, Centre Front, Right Front, and Surround) into a 2-channel stream (like on a video tape or an optical film). When you played back the 2-channel signal, it could be “decoded” into the original four channels. This system wasn’t perfect because of the encoding system. So, if a signal was in the centre channel, it was also showing up (albeit a little quieter) in the Left and Right Front channels. The same was true of the Surround signal – it also bled into the Left and Right Front channels. However, it was better than nothing, so it saw a wide distribution. In fact, it’s still possible to buy DVD’s like this one that are encoded in the original Dolby Surround format.
5.1 channels – surround sound (aka multichannel audio): Most movies released in the past few years on DVD have been mixed and distributed in 5.1 Surround Sound – but what, exactly does “5.1” mean? Well, the “5” part means that there are five main audio channels. these are the Left Front, Centre Front, Right Front, Left Surround and Right Surround channels. Whether or not you use 5 loudspeakers to reproduce these channels is up to you. For example, if you are using a BeoVision 11’s internal loudspeakers as the centre channel, then you are sending the Centre Front signal to two internal loudspeakers – just like the “dual mono” case I talked about above. Some people have really big home theatres, so they need more than one loudspeaker for each of the two surround channels – just like in a real movie theatre.
That leaves us with the “.1” which is the short-hand way of saying that there is an extra audio channel in the signal called an “LFE” channel (it stands for “Low Frequency Effects” or “Low Frequency Enhancement” depending on who you ask…) The reason we say it is only 0.1 of a channel is because it usually contains about one tenth of the frequency range of the other channels.
It’s important to note here that 5.1 channel systems are not like the Dolby Stereo system I talked about above. 5.1-channel systems are always “discrete” (and not necessarily “discreet”) meaning that if audio is supposed to be in one channel, it doesn’t bleed to adjacent channels. In other words, something in the Left Front channel is only in the Left Front channel and nowhere else.
It’s also important to note that the “LFE channel” and the “subwoofer channel” are not necessarily the same thing – so you should be careful not to say “LFE” if you mean “subwoofer” and vice versa.
It is also important to note that, if you are listening to a music-only 5.1 recording, you should turn off the LFE channel. Multichannel music recordings are almost always recorded, mixed, and mastered in 5.0 channels – no LFE. However, the business people that run the record labels don’t want to hear complaints from customers that are worried that their subwoofer isn’t working with their new disc, so they tell the mastering engineer to bleed a little bass to the LFE channel just to stop people from complaining. Since the only people who want something on the LFE channel are people with degrees in law, marketing, and business (and have no experience whatsoever with music or recording), there is absolutely no good reason to listen to it. This is why there is a menu item on the BeoVision 11 and BeoPlay V1 that says “LFE Input – On/Off” – it allows you to shut down the LFE channel input if you’re listening to a multichannel music recording.
It is also interesting, but less important to note here that not all audio formats are stuck with the low-frequency limit on the range of the LFE channel – in other words, in some systems (like SACD, DVD-Audio, Dolby TrueHD, and DTS HD-Master Audio, for example) the LFE channel can be used for more than just low frequencies, which brings us to…
6.0 channels – surround sound (aka multichannel audio): There are a small number of recordings, almost all of them music recordings on SACD (like this one), that are ostensibly in 5.1, but are actually in 6.0. This is because the recording engineer knew that:
- you shouldn’t use the LFE channel for music recordings
- the LFE channel in the distribution format (in this example, an SACD) has a full frequency range
So, in these cases, the LFE channel was used for capturing a sixth full-range audio channel instead of just a low-frequency channel. Typically, that sixth channel was used for height information, so the “correct” way to hear the recording is to send that audio channel to a loudspeaker above the listening position, preferably in or near the ceiling. This is why there is a menu item on the BeoVision 11 and BeoPlay V1 that says “LFE Input To Ceiling – On/Off”. It takes the LFE audio input channel and re-labels it a Ceiling channel so that it is re-routed to the appropriate loudspeaker. Of course, if you don’t route this audio channel properly, you probably just get a little something coming out of your subwoofer, and nobody is going to complain about that, as anyone with a degree in law, marketing, or business will tell you.
6.1 channels – surround sound (aka multichannel audio): There are a small number of movies that were released in systems that sort of combined the 5.1-channel discrete system and the “encoding” (more accurately called “matrixing” but we won’t get into that) system like the one used in the Dolby Stereo system. In these cases, the Left Surround and the Right Surround are used to contain a Centre Surround channel. So, you get a 6-channel system which fixes the problem that some people notice where the two surround channels don’t hang together very well… There is at least one fully discrete 6.1 system which is basically the same, only better.
7.1 channels – surround sound (aka multichannel audio): In the past couple of years, some of the really big-budget movies have been released in 7.1-channel surround sound – this is pretty easy to do because both Blu-ray and HDMI can handle it. This should make everything better, but, sadly, it’s where things start falling apart.
In theory, when we say “7.1 surround”, I know what you mean, and vice versa – and in most (possibly all) cases, that might be true. A “standard” 7.1 loudspeaker configuration is basically the same as the 5-channel configuration with a Left Back and Right Back loudspeaker added. The result is shown in the figure below.
The problem is that this is not the only possible version of 7.1. In fact, there are at least seven different variants of 7.1. Including the one above, these are as follows:
- Standard: Centre Front,L and R Front, L and R Surround, L and R Back, LFE
- Front Wide: Centre Front, L and R Front, L and R Front Wide, L and R Surround, LFE
- Front Height: Centre Front, L and R Front, L and R Surround, L and R Front Height, LFE
- Centre Height: Centre Front, L and R Front, L and R Surround, Centre Back, Centre Front Height, LFE
- Centre Overhead: Centre Front, L and R Front, L and R Surround, Centre Back, Ceiling, LFE
- Side Height: Centre Front, L and R Front, L and R Surround, L and R Surround Height, LFE
- Rear Surround: Identical to the Standard configuration but with a slightly different loudspeaker placement (with the surround and back loudspeakers placed closer together).
So, we have a problem. Just because a Blu-ray is encoded in 7.1 doesn’t necessarily mean that the channel allocations or the loudspeaker positions are the same from disc to disc.
More than 7.1 audio channels: To infinity and beyond! Believe it or not, there are some formats and systems that have more than 7.1 audio channels
- 9.1 channels: This format can be found, for example, in Dolby ProLogic IIz-encoded materials
- 10.2 channels: This format is built on the 5.1 standard, and includes 5 more main channels (including two dipole “diffuse radiators”) and an extra LFE channel. It was originally proposed by Tomlinson Holman
- 11.1 channels: This format is seen, for example, in the DTS Neo:X system
- 22.2 channels: This format is an experimental one built, in part, by Kimio Hamasaki at NHK
- Dolby Atmos – which doesn’t really have a set number of channels (although it can handle playback systems with up to 64 loudspeakers) and is only available in theatres
- A monster called Wavefield Synthesis, but that typically needs hundreds of loudspeakers to work reasonably well.
- and others
- and more to come (to quote Madonna’s character from Dick Tracy “More is better than nuthin’ – but nuthin’s better than more!”)
So, let’s assume that anything between 1.0 and 7.1 channels of audio can arrive at the input of our television, since HDMI can currently support up to 8 channels of audio. Let’s now think about the output.
Loudspeakers: How many channels are going out?
Let’s say that you have just had your brand-new BeoVision 12 (with an equally new BeoSystem 4) installed. The BeoVision 12 is equipped with a single loudspeaker channel (although it has 4 loudspeaker drivers built-in – four 2″ midranges and one 3/4″ tweeter, each with its own dedicated ICEpower amplifier). We’ll also assume that you don’t have any external loudspeakers attached – you only have the internal single loudspeaker. This means that, no matter how many audio channels come into the BeoSystem 4 – from a 1.0 mono news program up to a 7.1 Blu-ray – you’ll expect to be able to hear everything you’re supposed to hear. This means that the BeoSystem 4 will usually have to mix more than one channel at its input down to one channel at its output (the BeoVision 12 loudspeaker) – a process called downmixing.
But what about if tomorrow, the truck from the B&O shop shows up and drops off 6 new loudspeakers and a subwoofer. You set up the loudspeakers as your Left and Right Front, Left and Right Surround, Left and Right Back and Subwoofer – all in the correct places like in the drawing above – and you sit down and turn on a CD. Now you have only two audio channels coming into the system, but you probably want to hear something coming out of all of those loudspeakers. This means that we have to do something to get signals to go to more than two loudspeakers – we have to upmix the signal from 2.0 to 7.1. In fact, since most of the things you’ll be listening to and watching will be 1.0 to 5.1 (not many movies are in 7.1 yet) – you’ll probably be upmixing most of the time.
Now let’s take a typical situation – you have a 5.1 loudspeaker system (5 main loudspeakers and a subwoofer) and you have input signals that can range from 1.0 up to 7.1. This means that, sometimes, when you have a 1.0 or a 2.0 input from a TV channel or a CD, you want to upmix to 5.1. Sometimes, when you have a 5.1 input, from a DVD, for example, you want the channels to go straight through unaffected. Sometimes, you’ll watch a Blu-ray in 7.1 and you want to downmix to your 5.1 output. And, of course, you don’t want to have to do anything for this to happen – it should just happen automatically.
And, then, there are the people who go big – they have a loudspeaker on every audio output of the BeoSystem 4 – so they have the BeoVision 12 plus 11 other loudspeakers. So, they’ll probably be upmixing everything all the time.
So, the moral of the story is that, as a manufacturer, we don’t know how many loudspeakers you have. The BeoSystem 4 and BeoVision 11 are not like a typical AVR that you buy at your local big-box electronics store. They are far more capable in many aspects…
Philosophy: What should an upmixer do?
Part 1: Behaviour
If you’re like me, you’re a realistic purist. This means that I firmly believe that, if two channels come into the input of the system, you should only have two loudspeakers playing those two channels – unless it sounds better doing it differently.
In other words, if I’m at home, alone, sitting in the “sweet spot”, perfectly located between my two front Left and Right loudspeakers, I want 2.0 in and 2.0 out. No mucking about.
However, if I’m sitting on the sofa with the family, not in the sweet spot, and I have use the “purist” configuration of 2.0 in and 2.0 out, things just don’t work. All of my phantom images will collapse into the closest loudspeaker and that’s that. So, in this case, if I can have an upmixer that can correct the situation and put the centre images back in the centre, whilst maintaining some spaciousness and width, I’ll turn it on.
However, not everyone is like me – not everyone is going to be turning an upmixer on and off according to which chair they’ve put their bum on. Most likely, most people will turn on an upmixer and leave it on (because they want to hear sound coming out of all the loudspeakers they’ve paid for).
So, in a perfect world, in my not-so-humble opinion: if you’re sitting in the sweet spot, and you turn the upmixer on and off, you should not hear any different whatsoever. However, if you’re not in the sweet spot, turning on the upmixer should improve things. (At least, as I said, that’s my philosophy. There are those who say that, even when you’re sitting in the sweet spot, an upmixer should enhance things like spaciousness and envelopment – but I disagree, since I’m a part-time purist…)
Part 2: Technique
So, let’s say that you’re faced with having to make an upmixer. You can basically do one of two things:
- Take the input, analyse the audio signal, decompose it into more audio channels, and send those to more loudspeakers.
- Take the input signal and create new audio channels – for example, feed it into a reverberation unit to make echoes and reverb that are not in the original recording.
The purist in me very firmly believes that an upmixer should not add anything to the original recording. It should derive components of the audio signal that are in the original recording and distribute them to the various loudspeakers. But it should NEVER add something that wasn’t in the original. So, no reverberation – no concert hall sound slapped on top of the original recording. (At least, that’s my philosophy. Some commercially available upmixers are built on the idea that they should do exactly this – for example, simulating a system where your stereo recording is played in a “good” listening room, and then a multichannel version of that simulation is played through your real system. In my opinion, this is misguided at best, since I don’t want the drums in the opening of Lyle Lovett’s song “Penguins” or the anchor on the evening news to sound like they’re being played in a bathroom.)
Bringing it all together
When we were designing the audio signal flow for the BeoSystem 4, BeoVision 11 and BeoPlay V1, we knew that we needed an upmixer. Believe it or not, the first question was not “can we licence one?” (in other words “what can we buy?”) – it wasn’t even “what does everyone else use?” The first question was “what do we need?” which turned out to be a very interesting question indeed!
We decided that we needed an upmixer with the following features:
- it has to understand all of the audio formats from 1.0 to all of the 7.1 variants (because we don’t know what you’re going to play)
- it has to be able to upmix from any of those formats to any other of those formats (because you should be free to set up your loudspeakers however you want)
- it has to be able to downmix from any of those formats to any other of those formats (because you should be free to set up your loudspeakers however you want)
- it has to be able to neither downmix nor upmix if the input format matches the output format
- it has to be able to switch seamlessly between downmixing, upmixing and through-putting automatically with changes in the signal (because you might switch from watching the news to watching a movie, either because you switched to watching a Blu-ray disc, or because the movie was the next thing coming on that TV channel…
- it has to allow some adjustment of some parameters by the end user (everyone likes a little salt and pepper now and again…)
- if you’re sitting in the sweet spot
- it should sound like it’s not doing anything, either spatially or timbrally (a fancy word for “tonal balance-ly”)
- if you’re not in the sweet spot
- it should improve the centre image location
- it should improve spaciousness
- it shouldn’t push the left and right images wider or narrower
- it shouldn’t mess up the timbre too much
Let’s just look at the second of those requirements, since that was a killer. What this means is that you, the customer, should be able to set up your loudspeakers using any current standard of loudspeaker configuration from 1.0 or 7.1 (all seven variants) and the upmixer should take care of everything for you. How can this be done? Well, our solution was to set about to make a master format that encompassed all of those standard formats. In other words, if I wanted to build a loudspeaker configuration in a (large) room that could be used to play back all of those formats (one at a time) – how many loudspeakers would I need and where would they have to be? The answer was 16 (although, I have to admit, a small part of me was hoping that the answer would be 42 – but I always want the answer to be 42…)
As you can see in the table above, we found that we need 16 channel allocations in order to be able to accommodate all of the standard surround sound configurations. Those of you with a BeoSystem 4, BeoVision 11, or BeoPlay V1 should recognise the master list of 16 allocations as the Speaker Role options in your menus.
As soon as we had that, we knew that we needed to build our own algorithm. So, I was given the job…
From there, it was more of an organic process than anything else. I locked myself in the listening room for a couple of months with a 16-channel system made of BeoLab 3’s (yes… I had 16 BeoLab 3’s running in a room that is only 6 m x 5 m x 2.5 m – there wasn’t much room for people to visit and chat) connected to a multichannel sound card connected to my Macintosh running Max/MSP and MATLAB. I started by inputting a 2.0 stereo signal and built an algorithm that derived signals from the stereo signal to send to the various loudspeakers – always making sure that, sitting in the sweet spot, I couldn’t hear the difference (too much…). However, sitting away from the sweet spot, adding the extra channels had to improve things. In many respects, this task was a lot like being a real tonmeister – but instead of taking a bunch of input signals from microphones in front of the orchestra or band, and mixing that down to two loudspeakers, I was taking two inputs (from a CD) and breaking it down into its constituent components for the various loudspeakers. Almost like reverse-engineering a recording.
Of course there were challenges. The system has to work for pop recordings (where every instrument is recorded in mono with a single microphone and the fake reverb is generated digitally) and orchestra recordings (where, at the opposite end, you have two microphones in a concert hall and nothing else) and everything in between. This took some tweaking – but that’s why it took a while to build. The other big challenge was ensuring that it works for more than just a 2.0 input – that it also behaves for other input formats.
In the end, we had an upmixer that had “grown” in Max/MSP. The problem then was how to implement it, since the televisions don’t run Macintosh software inside. The solution to this was reverse engineering – I took my Max Patcher and converted it to a flowchart that was given to our “real” programmers to write the DSP code that would live inside the final products. So, they wrote the code and we ran it on the early prototype of the television (which actually just looked like a raw screen and a printed circuit board on a piece of MDF, as you can see in the photo below…).
One of the “tricks” that I did when making the signal flow was to leave myself a bunch of parameters that I could tune after the whole algorithm was implemented. So, once the Max/MSP + Matlab version on my Macintosh was converted to software inside the final hardware, and that was verified to make sure the conversion was correct, I could start tweaking. This meant leaving the BeoLab 3’s in the listening room, but taking out the Mac and the sound cards and replacing them with an early television prototype (see the photo above… it was VERY early…). From there I could make minor adjustments in the upmixer’s behaviour, including the custom tuning of each step on each of the different sliders that are in the final menus (like “Frequency Tilt” and “Stage Width” – just to name two).
Finally, we had to make sure that the upmixing and downmixing worked with different combinations of inputs (from 1.0 to 7.1 signals) and output configurations (from one loudspeaker to 12, sometimes with subwoofers, sometimes not) and different loudspeaker types (mixing and matching different sets of BeoLab loudspeakers to make sure things held together even if you don’t have 7 BeoLab 5’s in your listening room).
The Wrap-up
Of course, this was the short version of the full story. The longer version would talk about how our first real version of the TrueImage upmixer in a commercial product was made for the first Advanced Sound System that we put in the Audi A8. It would also talk about how TrueImage isn’t a fixed algorithm – it’s constantly evolving to meet the needs of the product it’s put in. So it’s more like a toolbox of possibilities that we can work with in the development process of any of our products that have to negotiate a situation where the number of audio input channels isn’t equal to the number of output channels. This also means that, as time goes on, the TrueImage algorithm will certainly change to meet the needs of the signals that we can receive and the outputs that our products can deliver. However, that would be too much information for this week.