#22 in a series of articles about the technology behind Bang & Olufsen loudspeakers
Let’s say that you go to the store and you listen to a pair of loudspeakers with some demo music they have on a shelf there, and you decide that you like the loudspeakers, so you buy them.
Then, you take them home, you set them up, and you put one of your recordings, and you change your mind – you don’t like the loudspeakers.
What happened? Well, there could be a lot of reasons behind this.
Tip #1: Loudness
In the last article, I discussed why a “loudness” function is necessary when you change the volume setting while listening to your system. The setup of this article discussed the issue of Equal Loudness Contours, shown again as a refresher in Figure 1, below.

Let’s say that, when you heard the loudspeakers at the store, the volume knob was set so that, if you had put in a -20 dB FS, 1 kHz sine wave, it would have produced a level of 70 dB SPL at the listening position in the store. Then, let’s say that you go home and set the volume such that it’s about 10 dB quieter than it was when you heard it at the store. This means that, even if you listen to exactly the same recording, and even if your listening room at home were exactly the same as the room at the store, and even if the placement of the loudspeakers and the listening position in your house were exactly the same as at the store, the loudpspeakers would sound different at home than at the store.
Figure 2 below shows the difference between the 70 phon curve from Figure 1 (sort of what you heard at the store) and the 60 phon curve (sort of what you hear at home, because you turned down the volume). (To find out which curve is which in Fig 1, the phon value of the curve is its value at 1 kHz.)
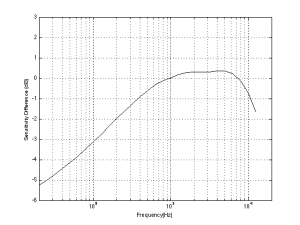
As you can see in Figure 2, by turning down the volume by 10 dB, you’ve changed your natural sensitivity to sound – you’re as much as 5 or 6 dB less sensitive to low frequencies and also less sensitive to the high end by a couple of dB. In other words, by turning down the volume, even though you have changed nothing else, you’ve lost bass and treble.
In fact, even if you only turned down the volume by 1 dB, you would get the same effect, just by a different amount, as is shown in Figure 3.

So, as you can see here, even by changing the volume knob by 1 dB, you change the perceived frequency response of the system by about 0.5 dB in a worst case. The quieter you listen, the less bass and treble you have (relative to the perceived level at 1 kHz).
So, this means that, if you’re comparing two systems (like the loudspeakers at the store and the loudspeakers at home, or two different DAC’s or your system before and after you connect the fancy new speaker wire that you were talked into buying), if you are not listening at exactly the same level, your hearing is not behaving the same way – so any differences you hear may not be a result of the system.
Looking at this a different way, if you were to compare two systems (let’s say a back-to-back comparison of two DAC’s) that had frequency response differences like the ones shown in Figure 3, I would expect that you might expect that you could hear the difference between them. However, this is YOUR frequency response difference, just by virtue of the fact that you are not comparing them at the same listening level. The kicker here is that, if the difference in level is only 1 dB, you might not immediately hear one as being louder than the other – but you might hear the timbral differences between them… So, unless you’ve used a reliable SPL meter to ensure that they’re they same level, then they’re probably not the same level – unless you’re being REALLY careful – and even then, I’d recommend being more careful than that.
This is why, when researchers are doing real listening tests, they have to pay very careful attention to the listening level. And, if the purpose of the listening test is to compare two things, then they absolutely must be at the same level. If they aren’t, then the results of the entire listening test can be thrown out the window – they’re worthless.
It’s also why professionals who work in the audio industry like recording engineers, mastering engineers, and re-recording engineers always work at the same listening level. This, in part, ensures that they have consistency in their mixes – in other words, they have the same bass-midrange-treble balance in all their recordings, because they were all monitored at the same listening level.
Tip #2: Recordings
If you were selling your house, and you got a call from your real estate agent that there were some potential buyers coming tomorrow to see your place, you would probably clean up. If you were really keen, not only would you clean up, but you would put out some fresh flowers in a vase, and, a half-an-hour before your “guests” arrived, you’d be pulling a freshly-based loaf of bread out of the oven (because there’s nothing more welcoming than walking into a house that smells like freshly-baked bread…) You would NOT leave the bathroom in a mess, your bed unmade, dirty dishes in the sink, and yesterday’s dirty socks on the floor. In short, you want your house to look its best – otherwise you won’t get people through the front door (unless the price is REALLY good…)
Similarly, if you worked in a shop selling loudspeakers, part of your job is to sell loudspeakers. This means that you spend a good amount of time listening to a lot of different types of music on the loudspeakers in your shop. Over time, you’ll find that some recordings sound better than others for some reason that has something to do with the interactions between the recordings, the loudspeakers, the room’s acoustics, and your preferences. If you were a smart salesperson, you would make a note of the recordings that sound “bad” (for whatever reason) and you would not play them for potential customers that come into your store. Doing so would be the aural equivalent of leaving your dirty socks on the floor.
So, this means that, if you are the customer in the shop, listening to a pair of loudspeakers that you may or may not buy, you should remember that you’re probably going to be presented with a best-case scenario. At the very least, you should not expect the salesperson to play a recording that makes the loudspeakers sound terrible. Of course, this might mean many things. For example, it might mean that the loudspeakers are GREAT – but if they’re being used to play a really bad recording that you’ve never heard before, then you might think that the reason it sounds bad is the loudspeakers, and not the recording. So, you’ll walk out of the shop hating the loudspeakers instead of the recording.
So, the moral of the story here is simple: if you’re going to a shop to listen to a pair of loudspeakers, bring your own recordings. That way, you know what to expect – and you’ll test the loudspeakers on music that you like. Even if you bring just one CD and listen to just one song – as long as the song is one that you’ve hear A LOT, then you’re going to get a much better idea of how the loudspeakers are behaving than if you let the salesperson choose the demo music. In a perfect reality, you’ll put on your song, and your jaw will drop while you think “I’ve NEVER heard it sound this good!”.
Tip #3: Room Acoustics
It goes without saying that the acoustical behaviour of a room has a huge effect on how a loudspeaker sounds (I talked about this a lot in this posting). So does the specific placement of the loudspeakers and the listening position within a room. (I talked about this a lot in this posting). So, this also means that a pair of loudspeakers in a shop’s showroom will NOT sound the same as the same loudspeakers in your house – not even if you’ve aligned the listening levels and you’re playing the same recording. Maybe you have a strong sidewall reflection in your living room that they didn’t have in the showroom. Maybe the showroom is smaller than your living room, so the room modes are at higher frequencies and “helping out” the upper bass instead of the lower bass. Maybe, in the showroom, the loudspeakers were quite far from the wall behind them, but in your house, you’re going to push the loudspeakers up against the wall. Any of these differences will have massive effects on the sound at the listening position.
Of course, there is only one way around this problem. If you’re buying a pair of loudspeakers, then you should talk to the salesperson about taking a demo pair home for a week or so – so that you can hear how they sound in your room. If you’re buying some other component in the audio chain that might have an impact on the sound, you should ask to take it home and try it out with your loudspeakers.
If you were buying a car, you would take it for a test drive – and you would probably get out of the parking lot of the car dealer when you did so. You have to take it out on the road to see how it feels. The same is true for audio equipment – if you can’t take it home to try it out, make sure that the shop has a good return policy. Just because it sounds good in the shop doesn’t mean that it’s going to sound good in your living room.
Tip #4: Personal Taste
I like single-malt scotch. Personally, I really like peaty, smoky scotch – other people like other kinds of scotch. There’s a good book by Michael Jackson (no, not that Michael Jackson – another Michael Jackson) that rates scotches. Personally, this is a good book for me, because, not only does he give a little background for each of the distilleries, and a description of the taste of each of the scotches in there – but he scores them according to his own personal ranking system. Luckily for me, Michael Jackson and I share a lot of the same preferences – so if he likes a scotch, chances are that I will too. So, his ranking scores are a pretty good indicator for me. However, if he and I had different preferences, then his ranking system would be pretty useless.
One of my favourite quotations is from Duke Ellington who said “If it sounds good, it is good.” I firmly believe that this is true. If you like the sound of a pair of loudspeakers, then they’re good for you. Any measurement or review in a magazine that disagrees is wrong. Of course, a measurement or a reviewer might be able to point you to something that you haven’t noticed about your loudspeakers (which may make you like them a little more or a little less…) but if you like the way they sound, then there’s no need to apologise for that.
However, remember that, when you read a review, that you are reading the words of someone who also has personal taste. Yes, he/she may have heard many more loudspeakers than you have in many more listening rooms – but they still have personal preference. And, part of that personal preference is a ranking of the categories in which a loudspeaker should perform. Personally, I divide an audio system’s (or a recording’s) qualities into 5 broad categories: 1. Timbral (tone colour), 2. Spatial (i.e. imaging and spaciousness), 3. Temporal (i.e. punch, transient response), 4. Dynamics (not just total dynamic range, but also things like short term “dynamic contrast”) and 5. Distortion & Noise. Each of these has sub-headings in my head – but the relative importance of these 5 qualities are really an issue of my personal preference (and my expectations for a given product – nobody expects an iThing dock to deliver good imaging, for example…). If your personal preference of the weighting of these 5 categories (assuming that you agree with my 5 categories) is different from mine, then we’re going to like different audio systems. And that’s okay. No problem – apart from the minor issue that, if I were a reviewer working for an audio magazine, you shouldn’t buy anything I recommend. I like sushi – you like steak – so if I recommend a good restaurant, you should probably eat somewhere else.
Of course, the fact that I will listen to different music played at a different level in a different listening room than you will might also have an effect on the difference between our opinions.
Tip #5: Close your eyes
This one is a no-brainer for people who do “real” listening tests for scientific research – but it still seems to be a mystery to people who review audio gear for a living. If you want to make a fair comparison between two pieces of audio gear, you cannot, under any circumstances, know what it is that you’re comparing. There was a perfect proof of this done by Kristina Busenitz at an Audio Engineering Society convention one year. Throughout the convention, participants were invited to do a listening test on two comparable automotive audio systems. Both were installed in identical cars, parked side-by-side. The two cars were aligned to have identical reproduction levels and you listened to exactly the same materials to answer exactly the same judgements about the systems. You had to sit in the same seat (i.e. Front Passenger side) for both tests, and you had to do the two evaluations back to back. One car had a branded system in it, the other was unbranded – made obvious by the posters hanging on the wall next to one of the cars. The cars were evaluated by lots of people over the 3 or 4 days of the convention. At the end, the results were processed and it was easily proven that the branded system was judged by a vast majority of the participants to be better than the unbranded system.
There was just one catch – every couple of hours, the staff running the test would swap the posters to the opposite wall. The two cars were actually identical. The only difference was the posters that hung outside them.
So, the vast majority of professional audio engineers agreed, in a completely “fair” test, that the car with the posters (which was the opposite car every couple of hours) sounded better than the one that didn’t.
Of course, what Kristina proved was that your eyes have a bigger effect on your opinion than your ears. If you see more expensive loudspeakers, they’ll probably sound better. This is why, when we’re running listening tests internally at Bang & Olufsen, we hide the loudspeakers behind an acoustically transparent, but visually opaque curtain. We can’t help but be influenced by our pre-formed opinions of products. We’ve even seen that a packing box for a (competitor’s) loudspeaker sitting outside the listening room will influence the results of a blind listening test on a loudspeaker that has nothing to do with the label on the box. (the box was a plant – just to see what would happen).
Tip #6: Are you sure?
One last thing that really should go without saying: If you’re doing a back-to-back comparison of two different aspects of an audio system, be absolutely sure that you’re only comparing what you think you’re comparing. For example, I’ve read of people who do comparisons of things like the following:
- sending a “bitstream” vs. “PCM” from a Blu-ray or DVD player to an AVR/Surround Processor
- PCM vs. DSD
- “normal” resolution vs. “high-resolution” recordings
If you’re making such a comparison, and you plan on making some conclusions, be absolutely sure that the only thing that changing in your comparison is what you think you’re comparing. In the three examples I gave above, there are potentially lots of other things changing in your signal path in addition to the thing your changing. If you’re not absolutely sure that you’re only changing one thing, then you can’t be sure that the reason you might hear a difference in the things you’re comparing is due to the thing you’re comparing. (did that make sense?) For example, given the three above examples:
- some AVR’s apply different processing to bitstream vs. PCM signals. Some use the metadata in a bitstream, and some players don’t when they convert to PCM. So, the REASON the bitstream and the PCM signals might sound different is not necessarily because of the signals themselves, but how the gear treats them. (see this posting for more information on this)
- Some DAC’s (meaning the chip on the circuit board inside the box that you have in your gear) apply different filters to a DSD signal than a PCM signal. Some have a different gain on the DSD signal (some “high resolution” software-based players also apply different gains to DSD and PCM signals). So, don’t just switch from DSD to PCM and think that, because you can hear a difference, that difference is the difference in DSD and PCM. It might just be your Equal Loudness Contours playing tricks on you.
- Some DAC’s (see previous point for my current definition of “DAC”) apply different filters to signals at different sampling rates. Don’t judge two recordings you bought at different sampling rates and think that the only difference is the sampling rate. The gear that you’re using to play the files might behave differently at different rates.
And so on.
A good analogy to this is to go to a coffee shop and buy two cups of coffee – one medium roast and one dark roast. While you’re not looking, I’ll add a little sugar to the dark roast cup – and I’ll bribe the person that made your coffee to make the medium one a couple of degrees colder than the dark one. You taste both, and you decide that dark roast is better than medium roast. But is your decision valid? No. Maybe you like sugar in your coffee. Maybe you prefer hotter coffee. Be careful how you make up your mind…
Summary
So, to wrap up, there are (at least) four things to remember when you’re shopping for audio gear:
- If you’re comparing systems, make sure that you’re listening at the same level.
- Always listen to a system using a recording with which you’re familiar – even if it’s a bad recording. Better something you know than something you don’t.
- Evaluate a system that you’re planning on buying in your own listening room.
- Don’t let anyone tell you what sounds good or bad. Ask them what they are listening to and for in a recording or a sound system – but decide for yourself what you like.
- If the listening test isn’t blind, it’s not worth much. Don’t even trust your own ears if you know what you’re listening to – your ears are easily fooled by your eyes and your pre-conceived notions. And you’re not alone.
- Be very sure that if you’re comparing two things, then the things you think you’re comparing are the only things that you’re comparing.